Methods
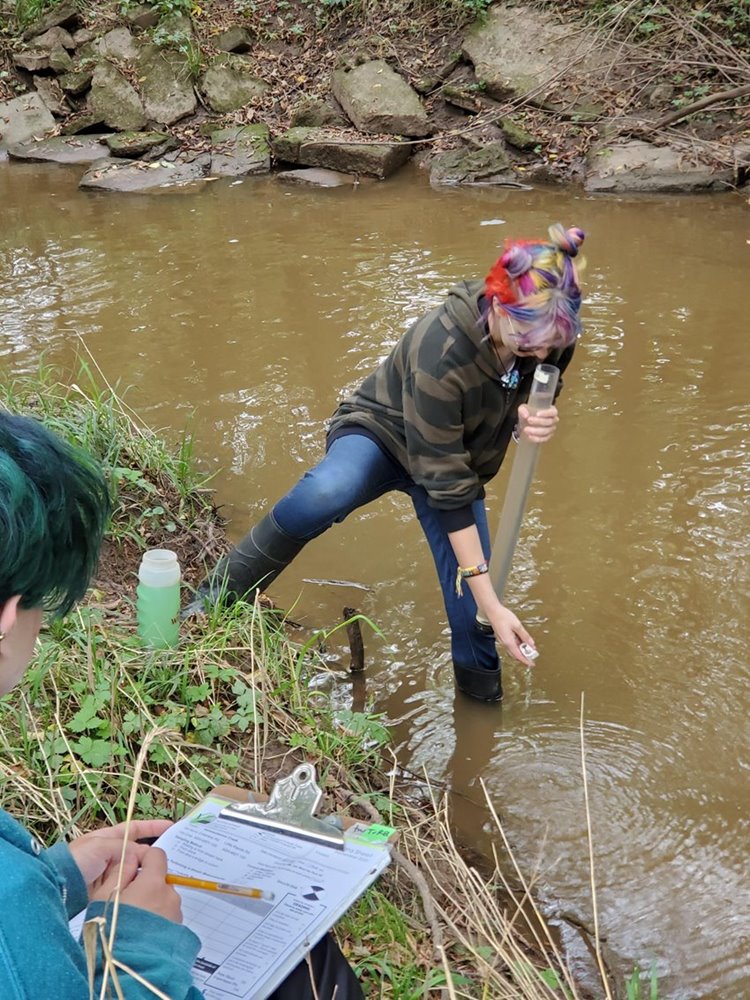
Authentic science learning happens when students literally become scientists as they explore the health of local streams in partnership with the scientific community. This collaboration creates a rich resource of information about water quality throughout the Fox River Basin. Scientists, municipal leaders, and students use this data to help inform decision-makers and stakeholders within our community.
Each student-teacher team performs seasonal rounds of monitoring at two or more monitoring locations within their selected stream. Sampling is done by all teams within the same 2-3 week period in spring, summer, and fall of each year. Most stream monitoring is performed when streams are wade-able and not affected by recent rain events.
Where do we monitor?
We monitor nine streams, two locations at each stream. stream. Seven streams are in the Lower Fox River Basin (Apple Creek, Ashwaubenon Creek, Baird Creek, Duck Creek, Dutchman’s Creek Wequiock Creek, and Trout Creek) Spring Brook, is located on the Upper Fox River and the Waupaca River is located in the Wolf River basin.
Who’s doing the monitoring?
Teachers and students from eight high schools complete all monitoring activities. Currently, 8 teachers and over 100 students are involved in the project. More than 1,000 students have participated in the program since 2003.
- Apple Creek: Appleton East High School
- Ashwaubenon Creek: West De Pere High School
- Baird Creek: Green Bay East High School
- Duck Creek: Green Bay Southwest High School, Green Bay West High School
- Dutchman’s Creek: Notre Dame Academy
- Spring Brook: Oshkosh Lourdes Academy High School
- Trout Creek: currently open
- Kankapot Creek: currently open
- Wapaca River: Weyauwega-Fremont High School
- Neenah Slough: Neenah High School
- Pigeon River: Etude High School
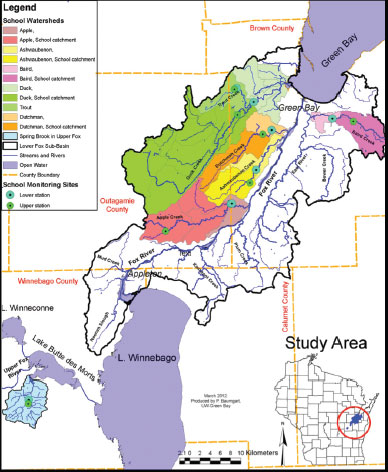
What do we measure?
The teams measure water quality parameters three times per year:
- stream flow (volumetric flow rate in cubic feet per second)
- temperature (affects the amount of dissolved chemicals, growth rates and survival of aquatic animals)
- dissolved oxygen (amount of oxygen available to aquatic animals)
- pH (acidity or alkalinity of the water)
- turbidity (amount of particles suspended in the water, like soil and silt)
- conductivity (amount of dissolved ions in the water, like salt)
- soluble reactive phosphorus (an important plant nutrient, but in excess it can cause huge algal blooms in the river and bay)
- ammonia and nitrate (an important plant nutrient, also harmful in excess)
We also measure items from the surrounding stream habitat once per year:
- macroinvertebrates (small organisms that live at the bottom of a stream, like insect larva, snails, worms and clams, indicators of stream health)
- plants and erosion along the stream corridor (determines habitat quality for wildlife and estimates buffering of run-off pollution into the stream)
- frogs and toads (amphibians populations are determined by listening to their breeding calls)
- birds (identified by sight and songs)
Definitions
Bird and Amphibian Monitoring*
In order to assess the biodiversity of the riparian community, bird and amphibian surveys were being performed as part of the LFRWMP. At a minimum of 10 stations along the riparian corridor, 10-minute bird counts are conducted on June mornings at daybreak to determine what species are using the habitat. Amphibian surveys are planned for three occasions in the spring as determined by overnight temperatures. Survey one occurs when overnight temperatures reach 41°F, survey two at 50°F, and survey three at 63°F. The time frame for amphibian surveys is from one-half hour after sunset to midnight. Audio CDs with detailed species’ calls provide the training for both the bird and amphibian assessments. *This monitoring ended in 2016.
Conductivity
Conductivity, or specific conductance, measures the ability of water to conduct an electrical current. A stream’s conductivity is directly proportional to the concentrations and types of positively and negatively charged ions present. Sources of ions are both naturally occurring and anthropogenic in origin, and include soil, bedrock, human and animal waste, fertilizers, pesticides, herbicides, and road salt. Specific conductance can also be used to approximate the Total Dissolved Solids (TDS) in the water.
A conductivity meter reads the conductivity directly from the stream. Conductivity is temperature-sensitive, but the meter automatically compensates for temperature differential by correcting values to a standard temperature of 25°C. The conductivity meter measures the flow of electrons over a specified distance, and is the reciprocal of resistance (ohms). Measurements are given in µS/cm (microSiemens per centimeter), which expresses the flow of electrons between two electrodes, each 1 square cm in surface area, that are 1 cm apart.
Streams in Northeast Wisconsin typically have conductivity values between 300 and 1800 µS/cm. During periods of snowmelt or in areas where there is barnyard runoff, the conductivity in a stream may exceed the detection limits for the Oakton ECTestr+ Low Conductivity Meter provided in the monitoring kits. In these situations, the conductivity should be reported as >1999 µS/cm. Although the State of Wisconsin has not established surface water quality standards for conductivity, it can be used as an indicator of excessive ion concentrations for further study.
Dissolved Oxygen
Unlike terrestrial environments, oxygen is typically a limiting factor in aquatic ecosystems. Dissolved oxygen (DO) concentrations are expressed as milligrams of oxygen per liter of water (mg/L). The amount of DO affects what types of aquatic life are present in a stream, because many species of fish and macroinvertebrates are sensitive to low DO levels. DO also regulates the availability of certain nutrients in the water. Many physical and biological factors affect the amount of dissolved oxygen in a stream.
The physical factors that influence DO are temperature, altitude, salinity, and stream structure. Temperature inversely controls the solubility of oxygen in water; as temperature increases, oxygen is less soluble. In contrast, there is a direct relationship between atmospheric pressure and DO; as the pressure increases due to weather or elevation changes, oxygen solubility increases. Salinity also reduces the solubility of oxygen in water. However, because streams in Northeast Wisconsin have relatively low salinity values, this factor is typically disregarded for our calculations. Stream structure also influences DO concentrations. Atmospheric oxygen becomes mixed into a stream at turbulent, shallow riffles, resulting in increased DO levels. Because there is less surface interaction between water and air in slow-moving water and deep sections of a stream, DO concentrations often decrease between surface and bottom measurements.
The biological processes of photosynthesis and respiration also affect dissolved oxygen concentrations in streams. As aquatic plants photosynthesize, they give off large amounts of DO during daylight hours. However, respiration from aquatic vegetation, microorganisms, and algae consume oxygen at all hours of the day and night. A stream experiencing an algal bloom exhibits large daily fluctuations in DO as extreme oxygen production during the day contrasts with the bacterial decomposition of algal detritus at night. Thus, the lowest concentrations of DO in the summer are typically observed just before dawn.
Biochemical oxygen demand (BOD) is another important factor that effects DO concentrations in streams. BOD is the amount of oxygen consumed by microbial decomposition of organic waste, and is measured by the change in DO in a sealed water sample over a five-day incubation period. High levels of organic pollution, such as that from sewage treatment plants, agricultural runoff, or industrial wastes, can significantly increase the BOD in a stream. Relatively healthy streams will have a 5-day BOD reading of less than 2 mg/L, whereas polluted streams may approach 10 mg/L.
DO must be measured directly in the stream, since concentrations change quickly once a sample is collected. A DO probe allows several measurements to be taken in a short period of time, allowing quick comparisons for different physical characteristics within the stream reach.
Streams in the Fox River Basin typically have dissolved oxygen values between 2 and 14 mg/L. The State of Wisconsin has set a minimum water quality standard of 5 mg/L DO as necessary for a stream to support fish and aquatic life. Trout streams may not have a DO level of less than 6.0 mg/L at any time, and may not have less than 7.0 mg/L DO during the spawning season.
Benthic Macroinvertebrates
The benthic (bottom-dwelling) macroinvertebrates supported by a stream are a great indicator of overall stream health due to their variable tolerance of pollution. Generally speaking, mayflies (Ephemeroptera), stoneflies (Plecoptera), caddisflies (Trichoptera), and riffle beetle larvae (Coleoptera) require a relatively pristine environment. Macroinvertebrates highly tolerant of pollution include midge larvae (Diptera), snails (Gastropoda), leeches (Hirundinea), and aquatic worms (Oligochaeta). Organisms such as scuds (Amphipoda), clams (Bivalvia), crayfish (Decapoda), cranefly larvae (Diptera), and aquatic sowbugs (Isopoda), are somewhat tolerant, and are found in a wide variety of water conditions.
Because macroinvertebrates are relatively immobile as compared to other aquatic organisms, they provide a quick snapshot of the condition of their surrounding habitat and the state of the stream’s food web. Macroinvertebrate samples are best taken from within a riffle because the increased level of dissolved oxygen available generally provides the most diverse population of the stream organisms. High diversity and numbers of macroinvertebrates indicate good water quality conditions, whereas presence of only pollution tolerant species or absence of macroinvertebrates suggests a degraded environment. Because naturally occurring coldwater and warmwater streams support different species of macroinvertebrates, researchers should not attempt to compare data from these two types of streams to each other in determining stream health.
Nutrients
Nutrients such as phosphorus and nitrogen are essential to maintain stream life. However, nutrient overloading into streams and lakes is one of the major challenges facing the Fox River Basin today.
A limiting nutrient is the nutrient which is in shortest supply in a particular ecosystem. Plant and animal growth will occur only to the point where that nutrient is no longer available. In freshwater systems, phosphorus is typically the limiting nutrient for plant growth, whereas nitrogen is the limiting nutrient in oceanic environments. Excessive amounts of these nutrients throw off the ecological balance of the aquatic system, with far-reaching impacts on biota. For example, phosphorus additions to Green Bay cause huge summer algal blooms. Similar conditions exist in the Mississippi River delta, except that it is nitrate causing phytoplankton blooms. Large amounts of detritus from these blooms settle to the bottom of the water column, where microbial decomposition depletes dissolved oxygen levels for fish and invertebrates.
Typical sources of excess nutrients in streams are wastewater treatment facility discharges and storm water runoff. Much progress has been made over the past 20 years in reducing phosphorus in wastewater, especially through the elimination of phosphates from laundry detergents. However, both manure and inorganic fertilizer inputs from agriculture and lawn-care are still widely uncontrolled nonpoint sources of nutrients. Fertilizers contain highly soluble compounds because plants need nutrients to be in solution for uptake by roots. However, these forms of nitrate, ammonia, and phosphorus are more able to migrate from the soil to nearby streams during precipitation events. In addition to fueling eutrophication in streams and lakes, both ammonia and nitrate can reach toxic levels under certain conditions.
pH
pH measures the hydrogen ion concentration of a solution, which controls whether a solution is acidic or alkaline. The pH scale is logarithmic, meaning that every one unit of change in pH is a ten-fold increase or decrease in acidity. The pH scale in the environment ranges from 0 (acids) to 14 (bases), with 7 considered neutral. pH must be measured directly in the stream, since changes in temperature can affect the pH value.
The pH of stream water is influenced by many sources. First, natural rainwater has a pH of approximately 5.6, attributable to mixing with carbon dioxide in the atmosphere to form carbonic acid. Nitrous oxides or sulfur oxides from fossil fuel consumption also can mix with water in the atmosphere to form acid rain, which can substantially lower the pH of streams. Acid rain may have more or less of an effect due to local geology. Calcium and magnesium ions dissolved from the surrounding limestone bedrock in the Fox River Basin increases the buffering capacity of the stream to resist changes in pH. However, volcanic bedrock weathers more slowly, so streams in igneous regions have less buffering capacity and acid rain has a greater impact on stream or lake chemistry. Photosynthesis also influences stream pH because carbon dioxide is used by that process during daylight hours and given off by respiration at night. Because carbon dioxide dissociates in water to create carbonic acid, decreased levels of CO2 during the day results in alkaline water conditions, and increased levels of CO2 at night create acidic pH values. This pH dynamic is most visible during algal blooms, with pH in some cases exceeding 9.0.
The optimum pH for most aquatic organisms is between 6.5 and 8.5. pH values outside this range may first affect reproductive processes, and then species survival. As pH controls the dissociation of substances into dissolved ions, low pH values increase the availability of certain toxic chemicals, such as mercury, lead, iron, chromium, and other heavy metals. Also, as pH increases, the ammonium ion (NH4) changes to ammonia (NH3), which is very toxic to aquatic life.
Streams in Northeast Wisconsin typically have pH values between 7.0 and 9.0, depending on the time of year that sampling occurs. The water quality standard for most surface waters in Wisconsin to support fish and aquatic life is a pH between 6.0 and 9.0, with no change greater than 0.5 units outside the estimated natural seasonal maximum and minimum values.
Because pH is measured on a logarithmic scale, to correctly calculate an average value for replicate measurements, the values would have to be transformed logarithmically into hydrogen ion concentrations, averaged, and then transformed back into pH. Rather than perform these calculations, the median pH measurement should be reported to the LFRWMP database. pH results measured with the pH Testr 3+ should be reported to 2 decimal places.
Riparian Habitat
Based on the EPA’s Stream Habitat Walk, the Lower Fox River Watershed Monitoring Program employs an easy-to-use approach for identifying the elements of a stream's habitat. Primarily a qualitative visual assessment, the habitat walk identifies potential water quality issues and allows observers to quickly become acquainted with their stream.
For the habitat walk, the stream is evaluated by its physical in-stream characteristics, local watershed integrity, visual biological observations, and an optional macroinvertebrate survey. Assessed in-stream characteristics include substrate composition, water appearance and odor, channel morphology, vegetation, and bank conditions. The local watershed is examined for types of land use present, and whether particular uses are impacting the stream water quality. Biological characterization includes the stream’s wildlife, fish, aquatic plants, and algae growth. Finally, an optional macroinvertebrate survey identifies basic invertebrates present and their relative abundance.
Streamflow
Streamflow, or discharge, is the volume of water in the stream flowing past a given point within a specific period of time. For the school-based monitoring program, streamflow measurements are recorded in cubic feet per second (cfs) for ease of comparison to the data reported by USGS.
Streamflow is calculated by multiplying the average width by the average depth by the average velocity by a bottom factor. To determine streamflow, a stream reach is selected that is at least 6” deep and fairly straight. The reach should contain few obstacles, and all the water should be flowing in a single channel (not braided). A run is typically a good selection for streamflow measurements because the water has sufficient speed and depth. Three transects across this stream reach provide the average width value. Along these three transects, equally spaced depth measurements are used to compute the average depth. Velocity is determined by the time it takes to float an orange or object of similar buoyancy the length of the stream reach. Oranges work well for measuring velocity because they float just below the water’s surface, where they cannot be influenced by wind. Finally, the stream bottom factor accounts for the friction of the water flowing past the stream substrate, with the assumption that average stream velocity is only 80% to 90% of the surface velocity. Rough bottom streams interspersed with submerged plants or rocks have slower water velocities as compared to smooth mud or bedrock. Thus, streams with cobble or gravel substrates or many aquatic plants have a bottom factor of 0.8, whereas streams with a smooth mud, silt, or bedrock substrate have a factor of 0.9.
The land use within a stream’s watershed greatly impacts stream discharge. Water enters the stream by direct precipitation into the channel, by surface runoff from the surrounding watershed, or from groundwater inputs. As a watershed becomes more urban, the increase in impervious surface cover causes less water to infiltrate to the groundwater and more surface runoff to enter the stream channel in a shorter period of time. Because of the reduced infiltration, the water table often drops and groundwater sources to the stream are reduced. This causes flashy conditions in which streams have higher peak discharges at the beginning of a precipitation event, and lower overall base flow conditions.
Streamflow has significant impacts on other water quality parameters. Fast-flowing, turbulent water at riffles increases dissolved oxygen levels through aeration. In contrast, low flow conditions typically result in higher water temperature and decreased oxygen levels. Periods of increased flow may result in greater turbidity, because the fast moving stream has enough energy to displace larger quantities and sizes of sediment particles. This erosive capacity is well illustrated by the exposed soil on the banks of a flashy urban stream. Finally, aquatic organisms are adapted to a variety of streamflow conditions based on feeding strategies, water temperatures, and dissolved oxygen concentrations. A diverse stream environment requires both slow and fast flowing habitats to support its biological community. Changes in streamflow dynamics, such as those brought on by an urbanizing watershed, can greatly impact the integrity of the stream ecosystem.
Temperature
Temperature is an important parameter in aquatic environments as it influences many aspects of stream physical, chemical, and biological health. Most aquatic organisms have limited optimal temperature ranges, which affect survival, spawning success, and metabolic rates. Annual temperature changes provide the stimulus for emergence of insects and spawning of fish. Because the high specific heat of water results in relatively slow rates of temperature change, aquatic species are buffered from the wide variations in temperature that terrestrial organisms are accustomed to. Therefore, large human-induced fluctuations in water temperature can have devastating impacts on stream biota. Increased water temperature also decreases the availability of dissolved oxygen, amplifies the stress caused to organisms by toxic compounds, and enhances algal and bacterial growth rates. Conductivity and pH are also influenced by the stream temperature, but most probes compensate for this effect.
The greatest source of heat to the stream is solar radiation. Streams with little riparian canopy cover are therefore warmer than shaded stream reaches. Deep streams are generally colder than shallow streams, as evaporation at the surface level slows heat transfer through the water column. Stream temperature is also impacted by groundwater, tributaries, rainwater, and discharge pipes. These inputs may either raise or lower the temperature, depending on season and source. Temperature also varies between different habitats within a stream reach, with backwater pools often warmer than the main stream channel.
Stream temperature can be measured with either a calibrated thermometer or with a dissolved oxygen, conductivity, or pH probe. Environmentally safe thermometers should be used to minimize the risks associated with potential mercury contamination. Data loggers which continuously record temperature measurements at set intervals may be used to show temperature fluctuations over time. For the LFRWMP, temperature measurements should be reported in °C to one decimal place.
Temperature readings for streams in the Fox River Basin typically are between 10°C and 20°C during our sampling periods. Wisconsin water quality standards state that there shall be no temperature changes that adversely affect aquatic life, and that daily and seasonal temperature fluctuations shall be maintained. By law, the temperature for warm water fisheries shall not exceed 89°F (31.7°C). A maximum temperature limit for cold water fisheries is not specified.
Turbidity / Transparency
The particulate matter carried by a stream determines its turbidity, or the relative muddiness or cloudiness of the water. Particulates in a stream consist of algae, sediment particles from erosion, coarse particulate organic matter (CPOM) such as leaves and twigs, and fine particulate organic matter (FPOM) that has been broken down by stream biota. Erosion is a natural geologic process. However, certain human activities such as farming, storm water discharge, and construction greatly increase the amount of erosion in a watershed. The increased sediment from these erosive activities blankets the stream bottom and destroys spawning areas and macroinvertebrate habitat. Sediment can also be resuspended into the water column by bottom feeders like carp or by walking through the stream. Suspended sediment blocks light needed by rooted aquatic plants, damages gills on fish and invertebrates, and decreases visibility for fish who must see their prey. Sediments can also carry adhered pollutants, such as heavy metals and phosphorus, into the stream.
There are several methods of measuring turbidity. The LFRWMP uses a transparency tube, which measures the depth at which a black and white crosshair pattern is visible at the bottom of a tube filled with stream water. Low transparency is highly correlated with high turbidity in streams. Another available method for measuring turbidity uses the Hach DR/850 colorimeter. This test reads the amount of light transmitted through the stream sample, and reports results in FAU, Formazin Attenuation Units. The most exact measurements of turbidity are made with a nephelometric turbidity meter. Turbidity meters report measurements in NTU, Nephelometric Turbidity Units, and have greater ability to determine lower levels of turbidity.
For the LFRWMP, measurements from the transparency tube should be reported in depth units of centimeters (cm). A transparency of about 25 to 35 cm is equivalent to about 25 NTU. A transparency of >60 cm is roughly equivalent to a turbidity of <10 NTU. A transparency of about 5 cm is roughly equivalent to a turbidity of about 200 – 300 NTU. A more detailed and robust relationship between turbidity and transparency for streams in Northeastern Wisconsin has not yet been developed.
Turbidity and transparency can also be related to total suspended solids and streamflow results for specific streams or rivers. Although general relationships have been reported, the relationship must be established on a site-by-site basis.